An introduction to what Organ-on-a-Chip technology is and why it’s setting the stage for the next generation of drug development and research.
To predict whether new therapeutics will be safe and effective for humans, drug developers first test them in animal- and cell-based models as well as computational tools that are designed to approximate human biology. Unfortunately, these approaches often fall short, as 90% of drug candidates that enter clinical trials fail, mostly due to a lack of efficacy or unforeseen toxicity. Not only is this inefficient system time- and resource-intensive, but it can be costly in terms of human lives1. To improve the odds of clinical success, researchers need models that better reflect human biology.
That’s where Organ-Chips come into play.
History of Organ-on-a-Chip Technology
In the early 2000s, researchers Dan Huh and Shuichi Takayama were interested in seeing whether they could model excess fluid accumulation, which occurs in diseased lungs, in an in vitro model. So, they created the first Organ-on-a-Chip system designed to mimic the airways of the lung2. Donald Ingber , M.D., Sc.D., from the Wyss Institute at Harvard, attended a presentation by Dr. Takayama and was amazed to hear the device make the same “crackle” sound he had learned to listen for to identify fluid in patients’ lungs—a phenomenon that remained poorly understood due to the inaccessibility of lungs for research.
Ingber invited Huh to his lab, and in 2010, they successfully created the first Lung-Chip capable of emulating breathing motions3. Following this success, Ingber and his team used funding from the Defense Advanced Research Projects Agency (DARPA), the Food and Drug Administration (FDA), and the National Institutes of Health (NIH) to develop more than 15 Organ-on-a-Chip models, including ones for the intestine, kidney, skin, bone marrow, and the blood-brain barrier. In 2014, Ingber founded Emulate to commercialize Organ-on-a-Chip technology.
Since then, Organ-Chips have progressed tremendously in quality, availability, and adoption Today, there have been over 100 publications using Emulate Organ-Chips, and the technology is used by 21 of the top 25 pharmaceutical companies as well as by academic research institutions and government agencies such as the FDA.
Stay Up to Date with the Emulate newsletter
How Does Organ-on-a-Chip Technology Work?
Organ-Chips combine cell culture with microfluidics to emulate the biological forces of different organ tissues and/or disease states, such as peristalsis in the intestines, breathing in the lungs, and blood flow through the vasculature4. The chips are flexible, thumb-drive-sized devices that contain parallel upper and lower microfluidic channels, each seeded with organ-specific cells. The channels are separated by a thin, porous membrane, which creates an interface for cell-cell communication (Figure 1). The membrane is coated with a tissue-specific extracellular matrix, helping to further drive tissue maturation.
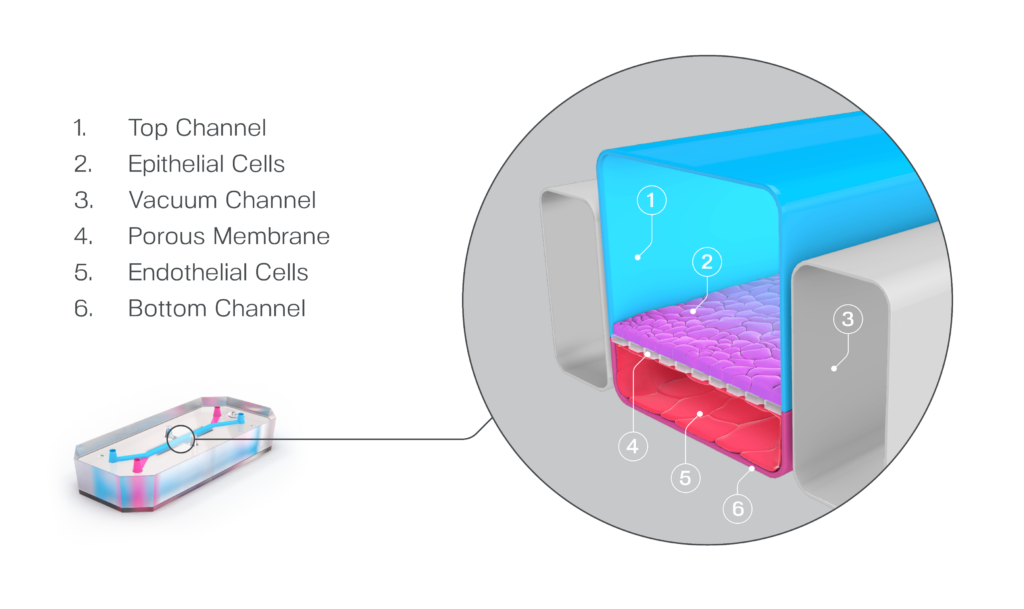
Organ-Chips are cultured in a microfluidic platform which automates the fluid flow and cyclic mechanical strain to create and maintain physiologically relevant conditions in the chip’s microenvironment. This ensures that the cells in the chip behave as though they were in their native organ—and react to drugs, chemicals, and other substances accordingly.
Researchers can collect data in real time with high-content microscopy imaging, effluent sampling, and a variety of functional measurements5. They can also perform traditional endpoint analyses such as cytotoxicity assays and immunohistochemistry as well as omics-based analysis to identify relevant disease pathways.
Organ-on-a-Chip Applications
Organ-on-a-Chip technology is used by academia and the pharmaceutical industry across a wide variety of areas, including toxicology, immunology, gene therapy, and cancer research. Within academia, Organ-Chips enable researchers to develop new models with closer-to-human gene expression to better understand human physiology and disease mechanisms. Within the pharmaceutical industry, the technology is primarily used to help determine drug candidates’ efficacy and toxicity ahead of clinical trials, helping to improve the quality of drugs that enter the clinic5 .
With a higher predictive validity than conventional models6, Organ-on-a-Chip technology can be used to identify drug candidates with a greater probability of success, improve safety for patients, lower development costs, and shorten the timeline for bringing new drugs to market. They can open new doors in medical research by allowing scientists to create new disease models and probe new biological pathways. Organ-Chips also hold significant promise in the field of personalized medicine, in which scientists could use the technology to create an in vitro model of a specific patient using biopsied cells and use that model to determine the best treatment strategy.
Advantages and Disadvantages of Organ-Chips
Compared to conventional culture and animal models, Organ-on-a-Chip technology provides several key advantages (Table 1). Traditional 2D cell culture models lack the structure, cellular heterogeneity, and physiological forces needed to emulate human biology, resulting in gene expression and morphology that poorly match in vivo tissue.
While organoid cultures provide 3D structure, they lack a dynamic physical microenvironment and endothelial co-culture, limiting human-relevant gene expression. Additionally, organoids exhibit highly variable ratios of cell populations, making it difficult to obtain robust and reproducible results. Animal models provide more physiologically relevant responses than conventional in vitro models but are limited in their predictive value by species differences, leading to poor clinical translatability. They also require considerable oversight and can pose ethical challenges7. For example, in cancer studies, successful translation of animal models to human clinical trials is less than 8%, which can cost a pharmaceutical company a large amount of money and prolong the search for lifesaving treatments8.
Table 1: Advantages and Disadvantages of Organ-on-a-Chip Technology 5,7,9
Advantages | Disadvantages |
Offers a tissue-specific dynamic microenvironment | Has less experimental longevity than animal models |
Contains human cells for greater human relevance than animal models | Has less throughput and scalability than 2D cell culture, limiting its utility in early stages of drug development |
Produces in vivo-like cell morphology | Requires more training and a more demanding workflow compared to traditional in vitro methods |
Uses multiple cell-types to better represent in vivo complexity | Is more expensive than conventional in vitro models |
Emulates human physiological processes such as breathing, peristalsis, and blood flow | Has less data acceptance criteria than conventional models from regulatory agencies |
Is less expensive than animal models | |
Reduces reliance on animal models | |
Can be used in precision medicine for personalized or group-specific treatment | |
Allows for real-time, functional, and traditional endpoint analyses |
As with any technology, Organ-Chips have some limitations; however, scientists are making rapid advancements with the technology, which will see fewer disadvantages as it matures.
Organ-on-a-Chip Technology: Future Prospects
Organ-Chip research and development are heading into the next decade with a plethora of exciting advancements and promising prospects. One such development is the passage of the FDA Modernization Act 2.0, which was signed into law by President Biden in December 2022. This legislation removes the requirement to use animal studies for investigating drug safety and efficacy and authorizes the use of alternatives, including Organ-Chips and other microphysical systems, when submitting new drugs for approval to the FDA. The law also includes funding to help create new Organ- Chip systems and further improve existing ones10. This will help accelerate the development and standardization of more Organ-on-a-Chip technologies, making it easier for pharmaceutical researchers to integrate them into their drug development pipelines.
Organ-on-a-Chip technology is also making exciting progress on the validation front . In the first-of-its-kind study released in December 2022, researchers showed that the Emulate human Liver-Chip meets the IQ MPS Affiliate’s qualification guidelines for use in predictive toxicology applications. When tested against a set of 27 toxic and non-toxic drugs, the Liver-Chip was found to have 87% sensitivity and 100% specificity6. Importantly, all of the toxic drugs used in the study were falsely deemed safe by animal testing, meaning the Liver-Chip has the potential to reduce drug-induced liver injury by up to 87%. Economic modeling in that study also showed that incorporating Organ-on-a-Chip technology into drug development programs can generate billions of dollars annually for the small–molecule pharmaceutical industry.
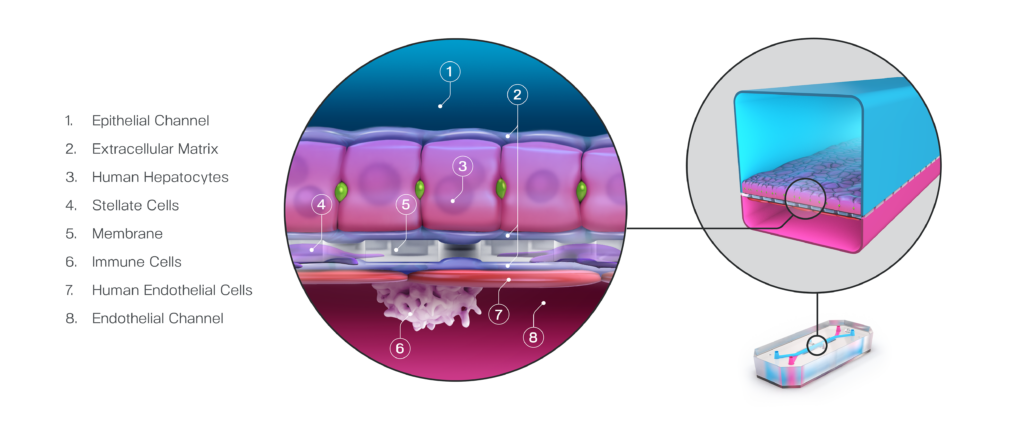
If this is what one Organ-Chip model is capable of, what happens when several are combined? Some researchers are looking to develop multi-Organ-on-a-Chip systems, incorporating two or more different Organ-Chip models to study interactions between organs or to model pharmacokinetics and pharmacodynamics7,11. One approach is to enable the interaction of multiple, independent Organ-Chip models through the transfer of cell media and effluent between chips. Ultimately, the goal would be to create Human-on-a-Chip system that emulates the response of the entire human body. A system like this could render animal models obsolete, further optimizing the drug development process12.
Of course, the potential for Organ-Chips is not bound to any one purpose—nor to Earth itself. With space exploration becoming more accessible, there is an increased need to understand the effects of space travel on human biology. In collaboration with NASA and the consulting firm IRPI, researchers from Emulate sent Organ-Chips and a Human Emulation System to the International Space Station (ISS) to test the effects of space travel on astronauts13,14. This would ultimately enable researchers to predict how an astronaut’s body would respond to the stressors of space travel before launching them into orbit.
Conclusion
Organ-on-a-Chip technology is a powerful preclinical model that can give pharmaceutical, academic, government, and other scientists better insights into human biology and disease mechanisms. By precisely replicating the biology and function of an organ, Organ-Chips overcome many of the issues with traditional preclinical models, giving drug developers confidence in progressing drugs to human trials. Ultimately, this will help create more efficient drug development pipelines—and, most importantly, better, safer medicines for patients.
- Scannell, J. W. & Bosley, J. When Quality Beats Quantity: Decision Theory, Drug Discovery, and the Reproducibility Crisis. PLoS One 11, e0147215 (2016).
- Huh, D. et al. Acoustically detectable cellular-level lung injury induced by fluid mechanical stresses in microfluidic airway systems. Proc. Natl. Acad. Sci. U. S. A. 104, 18886 (2007).
- Huh, D. et al. Reconstituting Organ-Level Lung Functions on a Chip. Science (80-. ). 328, 1662–1668 (2010).
- Ingber, D. E. Human organs-on-chips for disease modelling, drug development and personalized medicine. Nat. Rev. Genet. (2022) doi:10.1038/s41576-022-00466-9.
- Leung, C. M. et al. A guide to the organ-on-a-chip. Nat. Rev. Methods Prim. 2022 21 2, 1–29 (2022).
- Ewart, L. et al. Performance assessment and economic analysis of a human Liver-Chip for predictive toxicology. Commun. Med. 2022 21 2, 1–16 (2022).
- Ingber, D. E. Is it Time for Reviewer 3 to Request Human Organ Chip Experiments Instead of Animal Validation Studies? Adv. Sci. 7, 2002030 (2020).
- Mak, I. W. Y., Evaniew, N. & Ghert, M. Lost in translation: Animal models and clinical trials in cancer treatment. Am. J. Transl. Res. 6, 114–118 (2014).
- Singh, D., Mathur, A., Arora, S., Roy, S. & Mahindroo, N. Journey of organ on a chip technology and its role in future healthcare scenario. Appl. Surf. Sci. Adv. 9, 100246 (2022).
- Sen. Paul, R. [R-K. S.5002 – 117th Congress (2021-2022): FDA Modernization Act 2.0. (2022).
- Bhatia, S. N. & Ingber, D. E. Microfluidic organs-on-chips. Nature Biotechnology vol. 32 760–772 (2014).
- Syama, S. & Mohanan, P. V. Microfluidic based human-on-a-chip: A revolutionary technology in scientific research. Trends Food Sci. Technol. 110, 711–728 (2021).
- Emulate, Inc. is Researching Human Biology With Tissue Chips in Space. https://www.issnationallab.org/emulating-human-biology-with-tissue-chips/.
- Low, L. A. & Giulianotti, M. A. Tissue Chips in Space: Modeling Human Diseases in Microgravity. Pharm. Res. 37, 1–6 (2020).